Quantitative, structural and molecular changes in neuroglia of aging mammals: A review
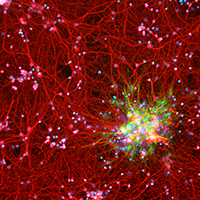
Accepted: 27 May 2021
HTML: 11
All claims expressed in this article are solely those of the authors and do not necessarily represent those of their affiliated organizations, or those of the publisher, the editors and the reviewers. Any product that may be evaluated in this article or claim that may be made by its manufacturer is not guaranteed or endorsed by the publisher.
Authors
The neuroglia of the central and peripheral nervous systems undergo numerous changes during normal aging. Astrocytes become hypertrophic and accumulate intermediate filaments. Oligodendrocytes and Schwann cells undergo alterations that are often accompanied by degenerative changes to the myelin sheath. In microglia, proliferation in response to injury, motility of cell processes, ability to migrate to sites of neural injury, and phagocytic and autophagic capabilities are reduced. In sensory ganglia, the number and extent of gaps between perineuronal satellite cells – that leave the surfaces of sensory ganglion neurons directly exposed to basal lamina– increase significantly. The molecular profiles of neuroglia also change in old age, which, in view of the interactions between neurons and neuroglia, have negative consequences for important physiological processes in the nervous system. Since neuroglia actively participate in numerous nervous system processes, it is likely that not only neurons but also neuroglia will prove to be useful targets for interventions to prevent, reverse or slow the behavioral changes and cognitive decline that often accompany senescence.
How to Cite
PAGEPress has chosen to apply the Creative Commons Attribution NonCommercial 4.0 International License (CC BY-NC 4.0) to all manuscripts to be published.
Similar Articles
- A. Vetuschi, A. D'Alfonso, R. Sferra, D. Zanelli, S. Pompili, F. Patacchiola, E. Gaudio, G. Carta, Changes in muscularis propria of anterior vaginal wall in women with pelvic organ prolapse , European Journal of Histochemistry: Vol. 60 No. 1 (2016)
- T. Karaca, F. Bayiroglu, M.U. Yoruk, S. Kaya, S. Uslu, B. Comba, L. Mis, Effect of royal jelly on experimental colitis Induced by acetic acid and alteration of mast cell distribution in the colon of rats , European Journal of Histochemistry: Vol. 54 No. 4 (2010)
- Qingjing Gao, Wenqian Xie, Wenjing Lu, Yuning Liu, Haolin Zhang, Yingying Han, Qiang Weng, Seasonal patterns of prolactin, prolactin receptor, and STAT5 expression in the ovaries of wild ground squirrels (Citellus dauricus Brandt) , European Journal of Histochemistry: Vol. 67 No. 4 (2023)
- Zbigniew Kmiec, Jacek Kieżun, Bartlomiej E. Krazinski, Przemyslaw Kwiatkowski, Janusz Godlewski, The role of galanin in the progression and prognosis of colorectal cancer: the unfinished story , European Journal of Histochemistry: Vol. 68 No. 1 (2024): 1954-2024: 70 Years of Histochemical Research
- M. Malatesta, C. Zancanaro, M. Costanzo, B. Cisterna, C. Pellicciari, Simultaneous ultrastructural analysis of fluorochrome-photoconverted diaminobenzidine and gold immunolabeling in cultured cells , European Journal of Histochemistry: Vol. 57 No. 3 (2013)
- G. Natale, E. Pompili, F. Biagioni, S. Paparelli, P. Lenzi, F. Fornai, Histochemical approaches to assess cell-to-cell transmission of misfolded proteins in neurodegenerative diseases , European Journal of Histochemistry: Vol. 57 No. 1 (2013)
- Andrea Amaroli, Sara Ferrando, Marina Pozzolini, Lorenzo Gallus, Steven Parker, Stefano Benedicenti, The earthworm Dendrobaena veneta (Annelida): A new experimental-organism for photobiomodulation and wound healing , European Journal of Histochemistry: Vol. 62 No. 1 (2018)
- Lucia Aidos, Luisa M. Pinheiro Valente, Vera Sousa, Marco Lanfranchi, Cinzia Domeneghini, Alessia Di Giancamillo, Effects of different rearing temperatures on muscle development and stress response in the early larval stages of Acipenser baerii , European Journal of Histochemistry: Vol. 61 No. 4 (2017)
- Manuela Malatesta, Transmission electron microscopy for nanomedicine: novel applications for long-established techniques , European Journal of Histochemistry: Vol. 60 No. 4 (2016)
- Francesca Bianco, Giulia Lattanzio, Luca Lorenzini, Chiara Diquigiovanni, Maurizio Mazzoni, Paolo Clavenzani, Laura Calzà, Luciana Giardino, Catia Sternini, Elena Bonora, Roberto De Giorgio, Novel understanding on genetic mechanisms of enteric neuropathies leading to severe gut dysmotility , European Journal of Histochemistry: Vol. 65 No. s1 (2021): Special Collection on Advances in Neuromorphology in Health and Disease
<< < 25 26 27 28 29 30 31 32 33 34 > >>
You may also start an advanced similarity search for this article.