Immunoreactivity and a new staining method of monocarboxylate transporter 1 located in endothelial cells of cerebral vessels of human brain in distinguishing cerebral venules from arterioles
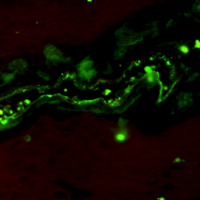
Submitted: 15 July 2021
Accepted: 8 September 2021
Published: 1 October 2021
Accepted: 8 September 2021
Abstract Views: 1007
PDF: 517
HTML: 13
Supplementary: 94
HTML: 13
Supplementary: 94
Publisher's note
All claims expressed in this article are solely those of the authors and do not necessarily represent those of their affiliated organizations, or those of the publisher, the editors and the reviewers. Any product that may be evaluated in this article or claim that may be made by its manufacturer is not guaranteed or endorsed by the publisher.
All claims expressed in this article are solely those of the authors and do not necessarily represent those of their affiliated organizations, or those of the publisher, the editors and the reviewers. Any product that may be evaluated in this article or claim that may be made by its manufacturer is not guaranteed or endorsed by the publisher.
Similar Articles
- Valentina Alda Carozzi, Chiara Salio, Virginia Rodriguez-Menendez, Elisa Ciglieri, Francesco Ferrini, 2D vs 3D morphological analysis of dorsal root ganglia in health and painful neuropathy , European Journal of Histochemistry: Vol. 65 No. s1 (2021): Special Collection on Advances in Neuromorphology in Health and Disease
- A.J.W. Pinto, I.F.G. de Amorim, L.J. Pinheiro, I.M.V.M. Madeira, C.C. Souza, H. Chiarini-Garcia, M.V. Caliari, W.L. Tafuri, Glycol methacrylate embedding for the histochemical study of the gastrointestinal tract of dogs naturally infected with Leishmania infantum , European Journal of Histochemistry: Vol. 59 No. 4 (2015)
- Anna Cleta Croce, Giovanni Bottiroli, Lipids: Evergreen autofluorescent biomarkers for the liver functional profiling , European Journal of Histochemistry: Vol. 61 No. 2 (2017)
- R. Pennati, A. Dell'Anna, G. Zega, F. De Bernardi, Immunohistochemical study of the nervous system of the tunicate Thalia democratica (Forsskal, 1775) , European Journal of Histochemistry: Vol. 56 No. 2 (2012)
- Flavia Carton, Laura Calderan, Manuela Malatesta, Incubation under fluid dynamic conditions markedly improves the structural preservation in vitro of explanted skeletal muscles , European Journal of Histochemistry: Vol. 61 No. 4 (2017)
- Lisa Marie Wadephul, Kathrin Arndts, Gnatoulma Katawa, Eva Dietlmeier, William Horsnell, Achim Hoerauf, Manuel Ritter, Walking a thin line between fixation and epitope binding – characterization of antigen retrieval methods suitable for eosinophil and HSV-2 staining in formalin-fixed female reproductive tissue , European Journal of Histochemistry: Vol. 68 No. 2 (2024)
- MA Croce, F Boraldi, D Quaglino, R Tiozzo, I Pasquali-Ronchetti, Hyaluronan uptake by adult human skin fibroblasts in vitro , European Journal of Histochemistry: Vol. 47 No. 1 (2003)
- I.C. Šoštarić-Zuckermann, K. Severin, M. Huzak, M. Hohšteter, A. Gudan Kurilj, B. Artuković, A. Džaja, Ž. Grabarević, Quantification of morphology of canine circumanal gland tumors: a fractal based study , European Journal of Histochemistry: Vol. 60 No. 2 (2016)
- Liqin Xi, Chen Wang, Pengyu Chen, Qi Yang, Ruiqi Hu, Haolin Zhang, Qiang Weng, Meiyu Xu, Expressions of IL-6, TNF-α and NF-κB in the skin of Chinese brown frog (Rana dybowskii) , European Journal of Histochemistry: Vol. 61 No. 4 (2017)
- Kentaro Nishida, Yuka Nomura, Kanako Kawamori, Akihiro Ohishi, Kazuki Nagasawa, ATP metabolizing enzymes ENPP1, 2 and 3 are localized in sensory neurons of rat dorsal root ganglion , European Journal of Histochemistry: Vol. 62 No. 2 (2018)
<< < 31 32 33 34 35 36 37 38 39 40 > >>
You may also start an advanced similarity search for this article.